A Cosmic Journey to Understand the Origin of the Complexity of the Universe
- Jerome
- Oct 8, 2015
- 16 min read
Whenever I look up and gaze at the night sky, I cannot help but feel awed by the sheer vastness and grandeur of space. Amidst my fascination with the heavens, I ask myself: what is the origin of this apparent complexity of the universe? This is truly a daunting question that has been bothering since time immemorial many other curious sentient beings aware of the continually evolving worlds around them. My meager answer to this question, however, sprang forth from the early memories of my Physics class I took last semester in the University of the Philippines. For good reason, we were tasked to create a computer program to simulate a phenomena exhibited in Conway’s Game of Life—a famous model in the field of cellular automata that features population dynamics of a given dummy ‘universe.’ In programming language, this universe is represented as an unbounded (toroidal) lattice or grid with each element or cell following simple rules that defines its state of being either dead (0) or alive (1), depending on the number of its adjacent cell(s). What’s remarkable with this is that while graphically simulating, I observed dynamic interaction among cells that drastically affected the population which made it unpredictable at any given time. Moreover, none of us expected that complex patterns would spontaneously emerge such that recurring figures that mimic the world came to life. After understanding the very deep implications of the concept at hand, I immediately knew that these apply not only to biological systems but also to the physical universe in general. The universe similarly originated from a basic irreducible form that evolved by following very simple and fundamental rules governing its behavior which gradually formed highly-organized structures and complex systems we see today.
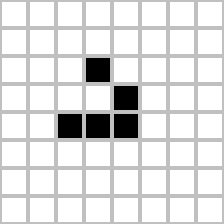
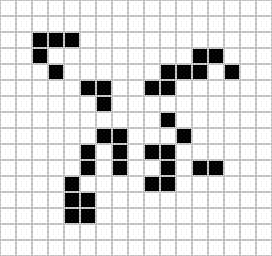
In my quest to solve the key problem posed however, I acknowledged that my original approach using cellular automatons is limited in many respects. Thus, I extended this concept using our recent knowledge in Biology, Chemistry, and Physics with emphasis in Cosmology relevant to answering the problem. Since the problem of origin of complexity of the universe is akin to a more fundamental problem concerning the origin and evolution of matter and hence universe itself, it is a requisite I believe to start answering the latter in order to shed light on the former.
What is complexity?

To begin with, it is important to objectively define what we mean by complexity. Unfortunately, complexity has no generally accepted definition that will hold true for any context in which it is asked. For example, how can one say that a human brain is more complex than the stock market? How do we then know one thing or process is more complex than another? To solve this conundrum, let us look closer at some of the things that we do know about complexity based on our knowledge in complex systems. First, we know that such systems involve many ‘simpler’ elements or agents that are strongly coupled. In other words, they are interacting nonlinearly in parallel with other different elements. Second, the manifestations of the seemingly unpredictable behavior being the spontaneous creation of new spatial, temporal, or functional structures are usually characterized by a coexistence of order and disorder. Third, complex adaptive systems are capable of adapting or evolving upon interaction with themselves and in its surroundings to generate qualitatively new collective behavior or emergent phenomenon as in the second. Thus, it is generally accepted that complexity increases with evolution. This becomes obvious when we compare the structure of higher form of creatures (e.g. animals, humans) to primitive life forms (e.g. bacteria, worms). Lastly, multi-scale description is crucial to understand complex systems.
Therefore, complexity can now be defined as a quality of a system that involves a hierarchy of description levels where the characteristics of a superior level emerge from those below it. I will use and later amplify this definition to describe the increasing complexity of the universe in the cosmological, geological, and finally anthropological viewpoint. The main hypothesis is that the universe strictly follows and maintains universal laws of conservation that governs its behavior. And the violation of such laws known as symmetry breaking is the origin of the complexity's growth across diverse evolutionary processes. This essay will delineate the hallmarks of the evolution of complexity from the beginning of the universe to its projected end in the future. In the process, I will determine the key stages of increasing complexity and the conditions necessary for such emergent complexity to occur. Moreover, I will argue that there exists a limit to the complexity of the universe on the basis of current cosmological models. This essay is not necessarily concerned with an anthropic universe since here it is also viewed how complexity is (possibly) evolving in other universes.
Although our universe can be very accurately described by simple equations for microscopic systems, the macroscopic level phenomena appear to be significantly different. This is especially true when we compare the structure and components of the universe at the moment of creation to that of the presently accelerating universe. To understand how complexity developed from then onwards, it is important to trace the history of the universe viewed in the framework of the standard model of particle physics, general relativity for gravity, and the standard model of Big Bang cosmology. Although several speculations still eludes us to truly probe the very early epochs near the Planck era, it is now well established that the early universe underwent a series of crucial evolutionary stages involving inflation, then baryosynthesis, followed by nucleosynthesis and finally recombination. These early epochs define the subsequent evolutionary stages of the universe whose main theme has been structure formation as discussed later.
Cosmological Evolution
Modern theories in particle physics predict that at very high energies, there exists a form of matter that creates a gravitational repulsion. Inflation then proposes that a patch of this primitive matter pictured as a bubble of some sort infinitesimally smaller than a fraction of an atom formed comprising the primordial universe. The gravitational repulsion exerted by this bubble was the driving force behind the Big Bang. Within this bubble of infinite density, pressure, and space-time curvature, the four known fundamental forces of nature: gravity, electromagnetism and the strong and weak nuclear forces were a combined “superforce.” Although extreme in these measures, the early universe was indeed regular (i.e. homogeneous and isotropic) in space. Then at temperatures of ~10^14 GeV, gravity spontaneously split off from the superforce as a result of symmetry breaking. The gravitational repulsion created by this bubble fueled its exponential expansion doubling in size every 10^-37 seconds while preserving the uniformity of the universe. The repulsive gravity material was unstable so it decayed ending the inflation. In the process of this "phase transition," it released energy to produce quark-gluon plasma as well as other elementary particles forming a hot, dense “primordial soup.” The temperature was still high such that the random motions of particles were at relativistic speeds, and particle–antiparticle pairs of all kinds were being continuously created and annihilated during collisions. The universe was still less than a second old when the electroweak force further decayed into separate electromagnetic and weak forces. As the universe continued to cool down, it generated the right conditions to form a soup of the building blocks of all baryonic matter: quarks and electrons, the constituents of the visible universe. The temperature was then no longer high enough to create new proton–antiproton pairs (and similarly for neutrons–antineutrons), so a mass annihilation immediately followed, leaving just a few of the original baryons and virtually none of their antibaryon counterparts. Roughly three minutes after the Big Bang, the temperature had decreased to a mere ~0.1 MeV which is cool enough for atomic nuclei to form. The free protons and electrons combined to make the light nuclei such as H which eventually fused to make He and so on with fixed abundances of approximately 75% H, 24% 4He by mass and the remaining small but important fractions of other light elements. About 380,000 years later when the temperature was ~0.3 MeV, radiation decoupled from matter and thus light traveled through the darkness after which the universe became transparent to radiation with the remaining photons constituting the cosmic background radiation as a relic light we still detect today.

From Simple to Complex
It is apparent that the universe has become more and more complex. The turning point of the apparent simplicity to complexity happened perhaps sometime midway between Big Bang and Baryogenesis. At some point when tiny quantum fluctuations in the density of “photon-baryon fluid” pervaded the early universe, the conservation of baryon number was violated which led to a very small excess of baryons (i.e. quarks and leptons) over antibaryons (i.e. antiquarks and antileptons). Elaborating this crucial event is important in the understanding of the origin of matter (and antimatter) content of the universe.
According to A. Sakharov, at least three conditions are required for Baryogenesis to occur, regardless of the exact mechanism. First, there must be a way of creating both more matter and antimatter particles of the kinds found in the present day universe—that is, those that make up the atoms we are made of. We believe that in the context of Grand Unified Theory (GUT) at very high energies such as that in the early universe, the interactions between elementary particles might as well violate the baryon number conservation. However, even at such conditions the universe could have still created the same number of each, and that wouldn’t cause a bias towards matter over antimatter. So a second condition is also necessary: there must be a selection mechanism not only to bias the creation of more matter than antimatter (Charge and Charge-Parity violation) but also to generate the excess number of the matter over antimatter. More importantly, in order to preserve the net number of baryons produced via the first two conditions, the early universe could not have been always in thermal equilibrium. This suggests that a third condition is necessary. That is, once we have an excess of matter over their antimatter counterparts, we must make sure that this excess is preserved even after annihilation as the universe continues to expand and evolve. The last actually is an out-of-equilibrium condition which tells us that as the temperature of the early universe dropped, it went through a phase transition, through which the particles consequently gained their mass. In analogy to water droplets in steam, droplets of the low temperature (massive) phase appeared within the high temperature (massless) phase, growing and coalescing, in a typical out-of-equilibrium phase transition. Since only in the high temperature phase are baryons created in excess over antibaryons, these excess baryonic particles will penetrate the droplets of the massive phase, becoming the net baryons in the low temperature phase. Finally as the droplets grow and coalesce, the whole universe is converted into the massive phase, completing the phase transition. This is roughly the present understanding of how the matter (and antimatter) and hence universe originated. From this we’ve gained tremendous insights on how complexity evolved from that crucial moment. But this is not the whole story.
Star and Planet Formation
Continuing our cosmic journey billions of years after the Big Bang, matter and gravity interacted to eventually form a typical molecular cloud of dust and gas in the slightly denser regions of the universe. In the process of gravitational collapse, it broke into smaller fragments releasing gravitational potential energy as heat. As the temperature and pressure increased, a fragment condenses into a rotating sphere of superhot gas that triggered thermonuclear fusion enabling the birth of a star. The onset of nuclear fusion leads relatively quickly to a hydrostatic equilibrium in which energy released by the core exerts a radiation pressure balancing the weight of the star, preventing further gravitational collapse. The star thus evolved quickly to a stable state, beginning the main sequence phase of its evolution. As these stars took shape, they produced the common heavier elements such as C, N and O until Fe in their stellar interiors. Depending on the mass of the star, it dictated its evolution to later stages. The more massive ones were destined to end in spectacular demise such as supernova. The stellar outflow from this cataclysmic event showered the heavier elements to the distant regions that eventually accreted into the newly-forming planets and perhaps into supermassive black holes harboring at the center of galaxies just like the Milky Way.

One of those planets, being in the Goldilocks’ zone, had conditions just right to allow water to build up in the atmosphere after being bombarded by eons of debris. Liquid water eventually gathered on the surface forming oceans and beneath it, mysterious chemical reactions ultimately formed primitive microbial life probably near volcano vents. Whenever a rich environment where atoms could come together to form complex chemical reactions, it ensured that Carbon atoms in particular would form complex organic matter such as amino acids, the precursors of life. Chemical catalysts sped up the reaction along whose products became the raw material for more complex chemical reactions. Eventually, a catalyst became an enzyme and amino acid chain became a protein. Then this loop of chemical reactions became metabolism and so Chemistry became Biology. Wherever the conditions were right for the development of hydrocarbons, liquid water and some form of self-replicating DNA, it seemed that life spontaneously formed in energy-sustaining regions. Any entities that could replicate by that time, and that had a variation both in their specific features and in their reproductive success, became the candidates for Darwinian natural selection and evolution.
The Unknown
To complete the picture, we also know that the cold dark matter model has become the leading candidate for the formation of structure in the Universe. Moreover, the universe today is dominated by the mysterious dark energy, which apparently permeates all of space. Merging our previous understanding together with the above models makes a clear prediction for the initial conditions for structure formation and predicts how structures grow hierarchically. When the universe was very young, it was likely infused with dark energy, but with less space and everything closer together, gravity had the upper hand and it was slowly breaking the expansion. But eventually after eons of years of expansion, the growing content of dark energy in the universe caused its expansion to slowly begin to accelerate. Roughly 13.75 billion years after the Big Bang, the universe is now 156 billion light years across. Our solar system has eight planets, more or less, in which the Earth is teeming with carbon-based life forms dominated by humans. These individuals that gradually developed the higher form of intelligence and consciousness are just beginning to realize how infinitesimally small specks they are in the grand scheme of things.
Biological Evolution
The rampant evolution of chemical and biological complexity portrayed above is primarily determined by two factors: natural selection and self-organization. Self-organization creates order in any complex system. Darwinian natural selection acts on this existing order and hones it further. But we know that symbiogenesis is not the only way in which new species can arise. Small changes in complex adaptive systems often can have unexpectedly huge consequences, including the emergence of new species. The effects of random changes (e.g. mutations) can gradually build up to a state wherein a sudden diversification (i.e. bifurcation) occurs and a new species arises. Speciation essentially may well be an emergent phenomenon.

General Pattern
We’ve gone a long way understanding the evolution of complexity by looking at the universe in a historical perspective. Nonetheless, the main idea just boils down to five key steps for complexity evolution to happen, as follows:
First, assume (though unlikely) that the universe was initially in a state of chaos—a collection of matter and energy lacking any organization.
Second, chaotic forces would cause matter and energy to interact in random ways.
Third, matter and energy will inevitably transform in such a way that a self-perpetuating pattern is produced.
Fourth, such self-perpetuating patterns that are fitted or best able to resist being broken by the chaotic forces tend to perpetuate themselves over cycles or generations leading to new structure formation or emergent phenomenon.
Last, chaos still imparts occasional variations in these patterns, thus causing “evolutionary experimentation.”
Based o
n the generalized conditions above, the implicit tendency of nature is toward repeating patterns. It would seem that the physical laws tend to organize matter and energy into patterned structures obeying principle of symmetry. Additionally, it is important to note the recurring fact of nature that she always tends to conserve a quantity. A connection between the two is best explained by Noether’s theorem. Noether's theorem simply states that for every symmetry there is a corresponding conservation law and vice-versa. By obeying the principle of least action and then applying Noether's theorem in a system in a symmetrical state, then a law of nature can be derived. Nature will always make sure that the Lagrangian, or the difference of kinetic and potential energies, is minimized. From this theorem, Newton's laws can be derived implying that the laws of nature is intrinsically symmetric.
As a short digression, this is the main reason why whenever physicists create a new theory, whether it addresses the origin of the universe, the interactions of quarks and other subatomic particles, or antimatter and so on, we first begin with the known symmetries that the system obeys. In fact, symmetries in Physics have been known to be the fundamental guiding principle in creating any new theory and hence understanding the nature of the universe. However, although ironic, the Physics becomes interesting when Noether’s theorem is violated. In fact, answers to the origin of mass and the matter-antimatter asymmetry problems emerge when Noether’s theorem is violated.
Symmetry-Braking
Apparently, the secret of nature is symmetry but much of the “texture” of the world is due to mechanism in symmetry breaking. In cosmic blueprint, P. Davies explains how complexity and self-organization can occur when dynamic systems are pushed beyond equilibrium with an open source of energy. As pointed out by I. Prigogine, this is actually a consequence of the laws of Thermodynamics. The second law itself is but a statement about the spontaneous breaking of symmetry translated in terms of dynamic “disequilibrium.” In particular, when a system is pushed away from a state of thermodynamic equilibrium by an influx of energy and/or matter, a gradient or asymmetry is created. If the departure from equilibrium is not too large, nature restores equilibrium by destroying the gradient. If the departure from equilibrium is sufficiently large, then the system is unable to restore the old configuration and as a result she must seek a new steady state or equilibrium state. For example, the Big Bang created an initial universe which contains an extremely high energy density and is sufficiently far from equilibrium. In order to reach an equilibrium state, the universe expanded rapidly, resulting in dramatic increase in entropy. During expansion and decrease in temperature, the initial unified symmetry broke apart into the subsymmetries of physical interactions, and the corresponding elementary particles were crystallized in stages leading to a more variety and complexity. It can compensate for the entropy decrease due to the formation of ordered structures such as stars, planets, galaxies and the even life on Earth. This is by the way one compelling argument that the Past Hypothesis, which posits that the universe has initial low entropy, is incorrect.
Indeed, there are also other several events crucial in the history of the universe that exactly portrayed out-of-equilibrium scenarios. The most important as pointed earlier is that when the universe was born as a quantum fluctuation. Baryogenesis violated the baryon number conservation as a result of spontaneous symmetry breaking, leading to a very small excess of quarks and leptons over antiquarks and antileptons. This resulted in the predominance of matter over antimatter which later amplified and became seeds of the large structures of galaxies and clusters of galaxies. As pointed out earlier as well, gravitational instability predominantly caused by dark matter and dark energy is a key ingredient in structure formation of galaxies and clusters of galaxies today. While in the context of everyday phenomena observed in Magnetism, Superconductivity and others, the dynamics is often shown as a manifestation of symmetry breaking. We know that the amino acids have an antisymmetrical carbon atom because it can occur in the left-handed configurations. Molecular antisymmetry seems to be a fact of life.
What’s more interesting however is when the departure from equilibrium is large, the system tends to find more efficient and commonly more complex ways of restoring symmetry. This then results in pattern formation and emergent phenomena or structures characteristic of complexity. As a typical example, the water molecules in ice crystals arrange themselves in increasing complexity every time during annealing to the freezing point. But does this mean that the overall complexity of the universe will continue to increase forever? My answer is no. There is no law of science which states that cosmic complexity in general must always increase. If we were to plot the “complexity curve” on a magnitude against the number of hallmarks graph, it will most likely look like a logistic curve that has a certain limit. After passing this point, it will in all likelihood decrease logistically as extrapolated in the paper by T. Modis.
Limit of Complexity of the Universe
There will come a time when the energy sustaining Sun, which is the major agent in driving terrestrial complexity of the in the planet, will cease to exist ~5 billion years from now. All life forms that are unable to sustain the harsh conditions will perish. Even though human civilization or some form of technologically advanced intelligent robots will likely flourish upon colonization in other distant star systems, they are no exception to the ultimate future of the universe—the Big Freeze. Over a time scale on the order of 10^14 years or less, existing stars will burn out and the stars will cease to be created such that universe goes dark. Over a much longer time scale, the galaxies will evaporate as the stellar remnants comprising it escape into space perhaps into black holes that will eventually evaporate via Hawking radiation. In some GUTs, the proton decay after at least ~10^34 years will convert the remaining interstellar gas and stellar remnants into positrons, electrons and photons. Some positrons and electrons will then annihilate into just pure energy leaving a universe that is in a high-entropy state consisting of a bath of particles and low-energy radiation.
Other Universes
We are limited by the observable universe that is one causal patch of a much larger unobservable universe. There are however parts of the universe which cannot communicate with us yet. Beyond our horizon, there may be worlds that have been developing some form of intelligent life as optimistically considered in the Drake equation. One compelling possibility is to consider that our universe, after extending it immensely far beyond our present horizon, as itself just one member of a possibly infinite ensemble of parallel universe. This multiverse concept, though speculative, is a natural extension of current cosmological theories, which have gained credence because they account for things that we do observe. Going back to the early universe when the false vacuum expanded as it decayed, the expansion could have been faster than the decay. This leaves the possibility that although only half of the false vacuum would remain after one half-life, it would be larger than the initial region. The false vacuum then could have never disappeared, but instead would continue increasing in volume indefinitely just as in inflation. The fragments of the false vacuum region would decay, producing new bubble universes at an ever-increasing rate. Our universe would be just one of the universes on this infinite tree of bubbles. The life forms might even be exceedingly intelligent that human beings having an advanced type III intergalactic civilization as M. Kaku envisioned. They might even have different values for the 6 dimensionless constants noted by Lord M. Reese (i.e. N≠1036, ε≠0.007, Ω≠0.3, λ≠0.7, Q ≠10^– 5, and D≠3) governing the structure of their own universe. Other kinds of space might not be three-dimensional, and they might alter the masses of elementary particles, or the forces that govern their behavior. If there are many kinds of space, the infinite tree of bubble universes would sample all the tantalizing possibilities. Beyond all these, however, cosmologists generally assume that the laws of physics are the same throughout this multi-bubble universe. We don’t really have any way of knowing, but our goal is to understand the consequences of the laws of physics as we know them, and not to idly speculate about other mythical worlds.
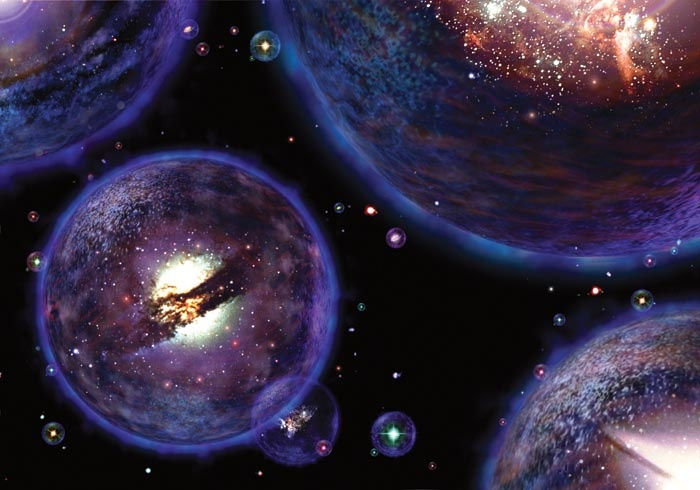
To momentarily end my quest in unraveling the roots of the complexity of the universe, let me quote what N. de Grasse-Tyson: We can know and we will know no matter how complex the universe is. We can know “because we are part of this universe, we are in this universe and perhaps most importantly than both of those facts—the universe is in us. This is the most astounding fact that I will forever remember every time I look at the night sky and contemplate the origins of the complexity of the universe.
Bibliography
A. K. Raychaudhuri, S. Banerji and A. Banerjee (2003). General Relativity, Astrophysics and Cosmology. New York: Springer.
Gross, D. J. (1995). Symmetry in Physics: Wigner's Legacy. Physics Today,. December
Hartle, J. B. (2003). Gravity: An Introduction to Einstein's General Theory of Relativity. San Franisco, CA: Addison Wesley.
Klaus Mainzer. (n.d.). Symmetry and complexity The Spirit and Beauty of Nonlinear Science. Springer.
Laughlin, F. C. (1997). A Dying Universe: the Long-term Fate and Evolution of Astrophysical Objects. Reviews of Modern Physics 69, #2, 337–372, April
Perepelitsa, D. V. (2008). Sakharov Conditions for Baryogenesis.
Nicolis, G., and Ilya Prigogine, 1977. Self-organization in Nonequilibrium Systems . New York: John Wiley & Sons.
Reese, M. (1999). Just Six Numbers: The Deep Forces that Shape the Universe. Great Britain: Weidenfeld and Nicolson.
Waldrop, M. (n.d.). Complexity The Emerging Science at the Edge of Order and Chaos.
Images
[1] http://i.livescience.com/images/i/000/064/447/i02/biological-hierarchy.jpg?1396421955
[2] http://lucidthoughts.com.au/wordpress/wp-content/uploads/2012/02/planetary-formation-950x1024.jpg
[3] http://cdn.phys.org/newman/gfx/news/hires/2013/bigbang.jpg
[4] https://equivalentexchange.files.wordpress.com/2012/05/noethers-theorem.jpg
[5] http://www.nature.com/naturejobs/2012/120906/images/nj7414-165a-i1.0.jpg
[6] https://s-media-cache-ak0.pinimg.com/736x/92/3b/53/923b5370ab04fb1490b6e64f0a879f4d.jpg
Comments